A technique known as nanotomography allows scientists to use x-rays, just like those used at the doctor’s office, to create a 3D image of extremely small structures within rocks or minerals. This ability to study rock samples at a resolution of 40 nanometers (human hair is around 75,000 nanometers thick!) helps scientists better understand underground chemical and physical processes that inform solutions to environmental challenges. EESA research scientist Harry Lisabeth is leading an effort to explore geological systems on this tiny scale to address fundamental questions about energy and the environment.
Many solutions to environmental challenges, such as capturing and storing atmospheric carbon dioxide (CO2) that heats the planet and generating and storing seasonal energy, can be developed by taking advantage of subsurface systems. However, because these solutions may involve fracture stimulation and fluid injection underground, they can place rocks under stress. To understand how this might impact underground rocks and reactions, EGD scientists are studying the interactions between rocks and fluids like CO2 on a very fine scale.
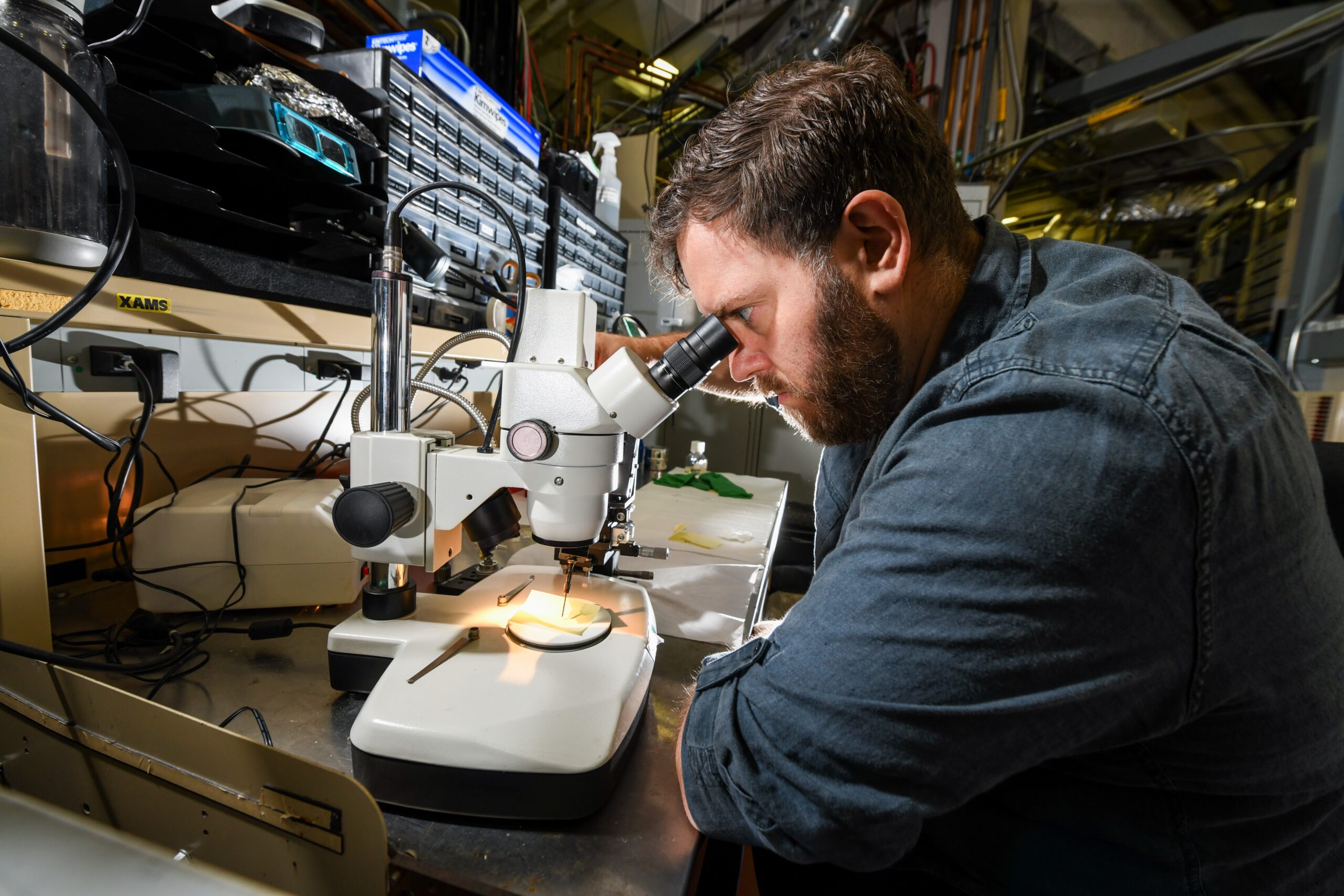
Lisabeth mounting samples for the nanotomography system. Photo Credit: Thor Swift
Lisabeth and his research team constructed an x-ray microscope that is used at Berkeley Lab’s Advanced Light Source (ALS) facility specifically for Earth Science applications. The microscope facility is housed within the ALS and supported and staffed through the Lab’s Basic Energy Science Fundamental Geoscience Program. The x-ray beamline works by taking hundreds of images of one rock sample from different angles. Researchers then use computational techniques to combine these images and reconstruct a 3D model of the particle. Because the system is dedicated to Earth Science projects, Lisabeth’s team can run complex experiments to see how these tiny rock samples respond in real time to changes in stress and chemistry.
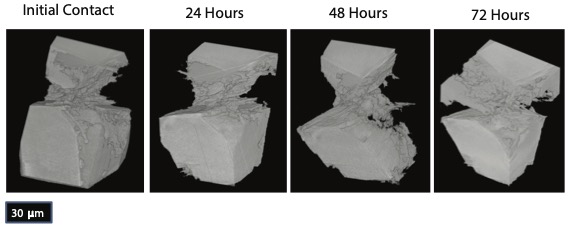
Snapshots from the beamline showing how calcite grains dissolve during a long-term pressure solution experiment.
“The overarching theme that ties it all together is fluid-rock interactions,” explained Lisabeth. “When a fluid passes through a rock it’s out of equilibrium with, reactions occur. That can lead to changes in mineralogy and physical properties. Whether it’s CO2 reacting with a basalt to form carbonate minerals, or water reacting with feldspar to form clays, changes in large-scale behavior are due to reactions happening between fluids and rocks. The nanotomography systems can image those reactions at a very fine scale.”
Changes in rock pore spaces, where most of the interactions between rocks and fluids occur, can lead to large shifts in the physical and chemical behavior of rocks – even at the subsurface reservoir scale. Lisabeth’s lab is particularly interested in studying the interaction of rocks with three fluids: CO2, hydrogen, and water.
CO2, a greenhouse gas, can exist in the atmosphere for 300-1,000 years – so simply reducing human greenhouse gas emissions might not be enough to combat the climate crisis. Researchers are now exploring how to remove CO2 from the atmosphere, but one critical question remains – where do we put it? EESA scientists are investigating how to store carbon dioxide in rocks, which offer great storage capacity since they occupy a large volume underground.
But before this solution can become a reality, researchers need to study the make-up of rocks and how minerals respond to CO2 injection. This is where the x-ray microscope comes into play; scientists are able to visualize the internal structure of a rock, nanoparticle by nanoparticle. They can also study how these particles interact with CO2, or with other particles themselves, to see what CO2 injection and stress might look like underground.
The lab also works to advance the potential of geological hydrogen energy as a carbon-free energy source. As some rocks interact naturally with water molecules to produce hydrogen gas, Lisabeth is studying this process on a fine scale, exploring how to speed up this chemical reaction and increase hydrogen production. Additionally, the x-ray microscope can help the research group understand how to safely and securely store hydrogen underground, addressing challenges with securing small hydrogen molecules that can escape rocks and clays easily.
This summer, Lisabeth is also helping to mentor a cohort of four student interns through a program started by California State University, Bakersfield, and Berkeley Lab called CAL–EPIC , in which students will analyze nanotomography data from the beamline to better understand the future of hydrogen storage in the Central Valley.
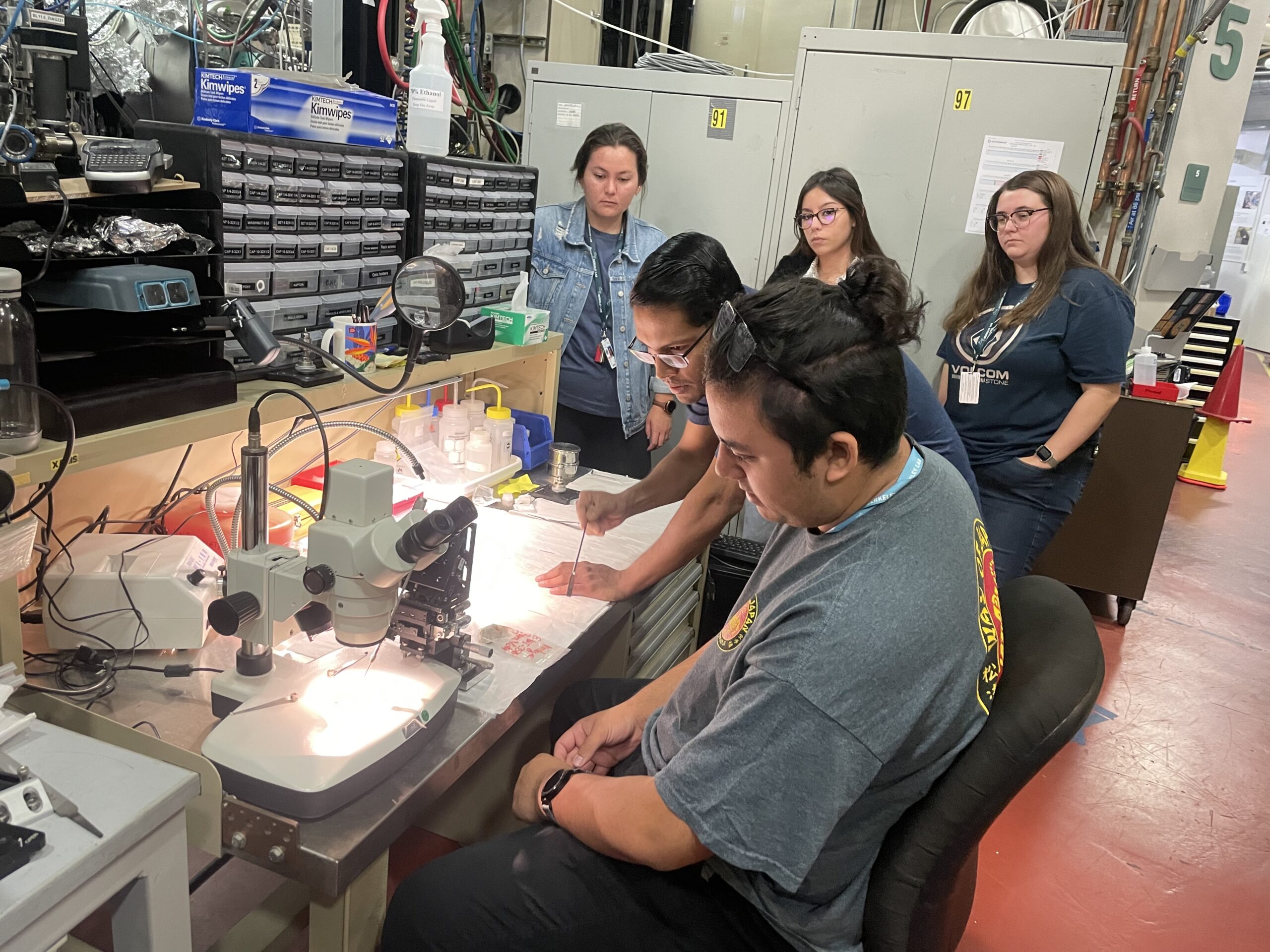
Berkeley Lab Postdoc Arun Bhattacharjee showing the CAL-EPIC interns how to mount samples for the nanotomography system. Photo courtesy of Harry Lisabeth
“Nanotomography is a great way of understanding nanoporous seals,” Lisabeth explained. “On this scale, we can see dynamic reactions that are happening when hydrogen moves through small pores, and study how to keep it stored for future energy use.”
Lisabeth is also using this method to study enhanced geothermal systems, which generate energy using the heat of hot liquid that flows underground. However, these systems don’t exist everywhere, which is why the research lab is using the x-ray beam microscope to explore on a very fine scale how to safely fracture rocks; this would allow water to flow through the hot rocks deep beneath the surface, creating more environments where these systems could generate carbon-free energy.
By harnessing the power of nanotomography, Harry Lisabeth and his team at Berkeley Lab are revealing the intricate dynamics of rock-fluid interactions and pioneering transformative solutions for pressing environmental and energy challenges. These research efforts can pave the way for innovative solutions regarding climate and energy—one tiny rock sample at a time.