The practice has shown since then that injecting large quantities of fluids into deep rock formations has the potential to provoke unwanted fault slip and seismic activity–and thereby trigger a potentially harmful leak of fluid into an aquifer or upwards towards Earth’s surface.
As part of global efforts to address climate change, EESA researchers who have been leading this type of research for decades are applying their expertise to investigating fault reactivation for the purpose of assessing safe long-term underground storage of carbon dioxide removed from the atmosphere. A new study by a team of EESA experts led by Yves Guglielmi is among the first to examine the impact of injecting fluids on “fault slip”–the movement of rocks on either side of a fault plane and on “fault leakage”–in initially very low-permeability and mechanically soft sedimentary rocks that contain a high percentage of clay minerals.
This research is among the first to show, based on field experiments and numerical modeling, how fluids are capable of leaking along an activated fault within a reservoir seal. Their paper was published in Nature Geoscience this month.
Because of their low permeability, these sedimentary rocks like shale form important geological seals above reservoirs in which to store carbon dioxide removed from the atmosphere underground using Geological Carbon Storage (GCS) technologies. GCS is essential to achieving carbon neutrality by 2050 but right now the quantity of carbon dioxide stored underground worldwide–40 megatons–would need to increase 150 times to meet this ambitious goal. Getting there requires addressing concerns over the safety of these technologies, not the least of which is the potential for leakage of CO2 once a fault is reactivated.
“It’s crucial to look at faults intersecting shales because their modes of activation and leakage remain poorly known compared to faults in reservoirs and in the deep rocks beneath sedimentary basins where most of the research focused during the last decades in relation to natural and induced seismicity,” said Guglielmi, a geologist and Berkeley Lab staff scientist whose research often takes him into underground rock laboratories such as the Mont Terri Rock Laboratory where this latest research was conducted in Switzerland.
Traditional wisdom is that a fault activates due to fault slip, but with this study, the researchers showed that it is different in the case of a shale fault. The team shows that slip triggers an initial increase in the fault permeability which is small but enough to allow for injected fluids to permeate the fault. After fluids have permeated the fault, a much larger opening (about a factor of ten larger than the initial slip-induced opening) is occurring.
An unexpected finding is that the part of the fault that slips and the part where fluid leakage occurs differ in size, the part of the fault that leaks being much smaller and much more “localized” than the part of the fault that slips. This is important because once a fault begins to slip it can be meters away from the location where pressure starts to build up and where a leakage flowpath is developing. This means that other types of monitoring methods may need to be developed to track leakage in shale rocks.
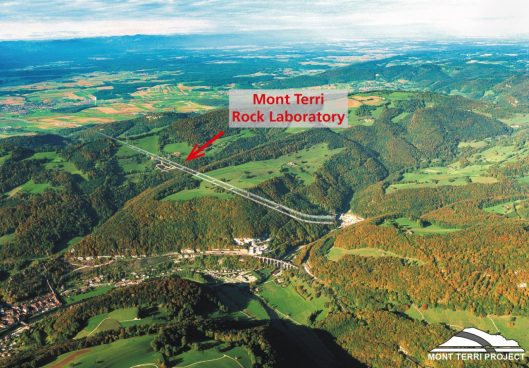
The Mont Terri Rock Laboratory is located in a suitable shale formation and provides a unique setting to conduct the relevant fault slip experiments at the appropriate scale, according to Guglielmi.
For these investigations, benchtop experiments conducted at laboratory scale (centimeter or less) are too small to allow researchers to draw any meaningful conclusions whereas field scale and reservoir scale–which span 100s of meters to kilometers respectively–are too vast to explore these research questions. However, in-situ experiments such as those that can be conducted at Mont Terri are at a scale big enough to integrate the full field complexity of a geological fault zone yet not so large as to be able to “control” the experiment’s boundary conditions.
Guglielmi and team were able to obtain very high-resolution measurements of the fault movements using a custom-built tool called SIMFIP (Step-Rate Injection Method for Fracture In-Situ Properties) which was designed specifically to examine what happens to rock fractures and faults when fluid pressure is applied. Using SIMFIP, water was injected into the fault in a highly controlled manner to generate seismicity significant enough so its impacts can be measured with very sensitive sensors and small enough such that no damage occurs in the underground research laboratory. The researchers also employed optical-fiber instrumentation that enables distributed acoustic sensing (DAS) or distributed strain sensing (DSS) capable of measuring strain–or how pieces of rock move in response to stimulation–across various intervals rather than between two points.
Their next steps, according to Guglielmi, are to see if this type of investigation can be replicated at a reservoir-caprock basin scale, and at industrial injection rates. Meanwhile, experiments at the Mont Terri site are far from being finished. In November, the team will go back to the site to inject CO2 into the fault, with the aim to compare if the fault response is the same as when injected with pure water. To our knowledge, it will be one of the first CO2 fault activation experiments worldwide.